Author: Francisco Cuenca-Fernández
Introduction and Methodology
Since the energy required to swim at a certain speed is derived from that specific speed (Santos et al., 2020; Zamparo et al., 2020), a training stimulus should also include exercises at race-specific velocity with the aim to stimulate different energetic pathways and aerobic power (Nugent et al., 2019). However, one of the problems of maintaining high-intensity exercises during training sessions of high-volume is the severe depletion of ATP and phosphocreatine stores (PCr) which results in excessive fatigue levels (Gorostiaga et al., 2010; MacInnis & Gibala, 2017). One appealing option to increase race-specific velocity training while obtaining the endurance performance gains, is a derivative of high-intensity training (HIT) known as Ultra-short race-pace training (USRPT) (Nugent et al., 2019). With this procedure, the aerobic and glycolytic systems are stressed through brief bursts of vigorous activity (e.g., 20 to 50 swimming intervals completed over short distances 15 to 100-m), interspersed with work-recovery ratios of 1:1, 1:2 or 2:1, according to the individual best competitive performance (i.e. competitive race-pace)(Laursen & Jenkins, 2002; Nugent et al., 2017). The application of this method may be supported by expected adaptations such as an increase in VO2max (MacInnis & Gibala, 2017), an improved ability of working muscle to produce and utilize ATP from the glycolytic system (Laursen & Jenkins, 2002), higher velocity at [La–] threshold (Nugent et al., 2017), and a decrease in the energy cost of swimming (-20%) (Zamparo et al., 2020).
In a counterbalanced crossover design, the metabolic (i.e., lactate), biomechanical (i.e., arm-strokes) and neuromuscular (i.e., CMJ) effects of fatigue between two swimming race-pace protocols were studied on 14 national competitive swimmers. One of the protocols consisted of 10 × 100-m swimming bouts (Race-pace training [RPT]), while the other consisted of 20 × 50-m swimming bouts (i.e., USRPT). In both protocols, swimmers were given individualized target times based on specific 200-m times and followed a work-recovery ratio of 1:1.
Results and Discussion
The results showed that both protocols achieved an intensity range within [La–] 8-12mM/L, but RPT produced higher [La–]max compared to USRPT. The [La–] values obtained in RPT (2 min: 10.8 ± 2.7; 5 min: 10.1 ± 2.6 mM/L) were higher than in USRPT (2 min:8.3 ± 2.7 [p = 0.021]; 5 min: 7.4 ± 2.8 Mm/L [p = 0.008]) and both were higher at 2 min compared to 5 min (p < 0.001) (Figure 1). Although USRPT and RPT set the same target intensities, the magnitude of [La–]max also depends on the duration of the exercise because glycolysis reaches near maximal rates after ~40-50s(Brooks, 2020), resulting in a greater impact on RPT.
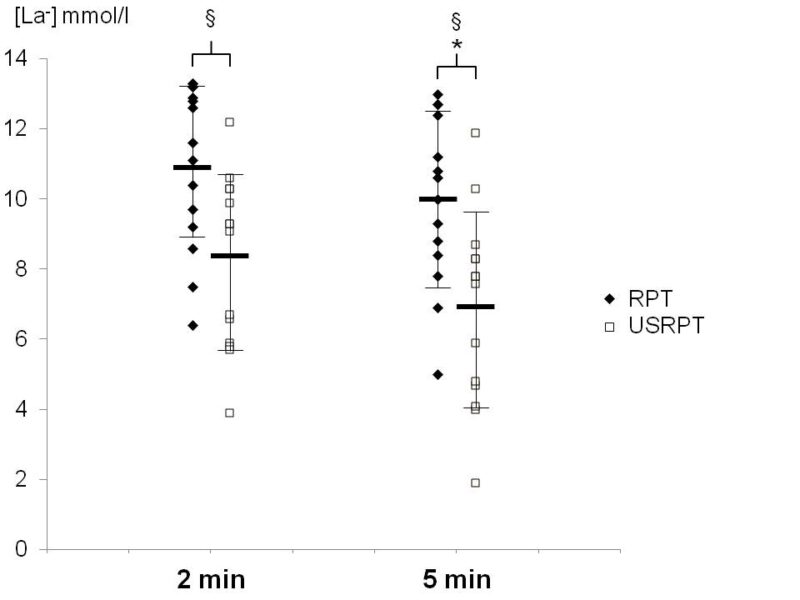
The total swimming time increased in RPT but remained more stable in USRPT (Figure 2A). Thus, a lower volume at race-pace intensity was achieved in RPT. The analysis conducted showed that increasing the number of arm-strokes entailed worse times in RPT (Males: r = 0.58; p < 0.001; Females: r = 0.64; p < 0.001), whereas this relation was not evidenced in USRPT (Males: r = 0.28; p < 0.001; Females: r = 0.10; p = 0.18). For a given distance and speed, a higher number of arm-strokes would represent a higher stroke-rate and a lower stroke-length and this could be related with a reduced capacity to generate propulsive impulse per stroke(Cuenca-Fernández et al., 2020; Santos et al., 2020), resulting in a higher energy cost(Zamparo et al., 2020).
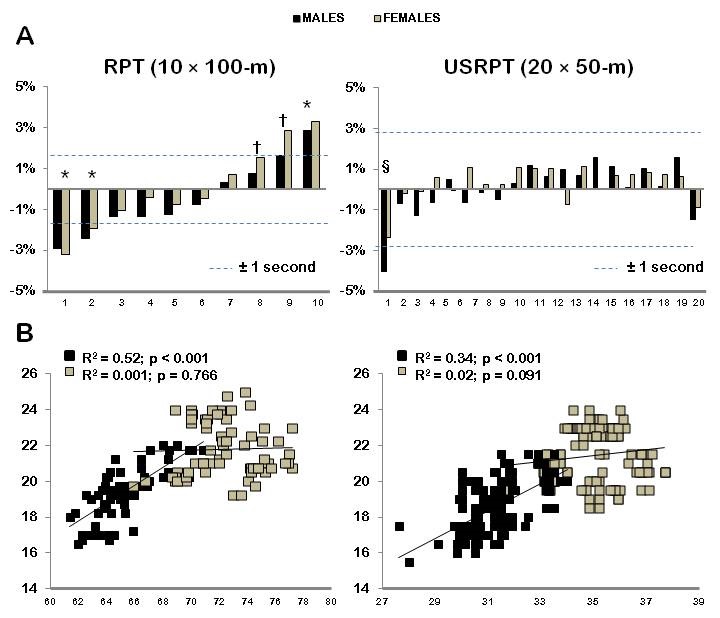
There was a deterioration in CMJ-height observed after both protocols at min 2. However, the CMJ-capacity was quickly restored at min 5 of recovery only in USRPT, with a trend (p = 0.07) for a CMJ-height potentiation. This is an important finding as a post-USRPT CMJ-height potentiation would be the result of the balance between fatigue and potentiation mechanisms(Boullosa et al., 2020). Hence, if fatigue had a direct force-depressing effect in muscles, this was possibly counteracted by other potentiation factors that increased force to the same extent after some minutes of rest (Cuenca-Fernandez et al., 2017).
Practical applications for coaches:
For a given training volume, USRPT is better than RPT to achieve more volume at race pace, maintaining the swimming patterns with a considerably lower metabolic and neuromuscular fatigue. Therefore, it is reasonable to suggest that increasing the frequency of USRPT training with lower metabolic stress and fatigue would allow athletes to accumulate more HIT volume at race-specific velocity. Similarly, RPT could be an interesting method for long-distance swimmers to create more stress to train [La–] tolerance.
Extended version of the study:
Cuenca-Fernández, F., Boullosa, D., Ruiz-Navarro, J. J., Gay, A., Morales-Ortíz, E., López-Contreras, G., & Arellano, R. (2021). Lower fatigue and faster recovery of ultra-short race pace swimming training sessions. Research in Sports Medicine, 1-14. Doi: 10.1080/15438627.2021.1929227
REFERENCES
Brooks, G. A. (2020). Lactate as a fulcrum of metabolism. Redox biology, 35, 101454.
Cuenca-Fernández, F., Ruiz-Navarro, J. J., & Arellano Colomina, R. (2020). Strength-velocity relationship of resisted swimming: A regression analysis.
Leave a Reply